Most metal corrosion occurs via electrochemical reactions at the interface between the metal and an electrolyte solution. A thin film of moisture on a metal surface forms the electrolyte for atmospheric corrosion. Wet concrete is the electrolyte for reinforcing rod corrosion in bridges. Although most corrosion takes place in water, corrosion in non-aqueous systems is not unknown.
Corrosion normally occurs at a rate determined by an equilibrium between opposing electrochemical reactions. The first is the anodic reaction, in which a metal is oxidized, releasing electrons into the metal. The other is the cathodic reaction, in which a solution species (often O2 or H+) is reduced, removing electrons from the metal. When these two reactions are in equilibrium, the flow of electrons from each reaction is balanced, and no net electron flow (electrical current) occurs. The two reactions can take place on one metal or on two dissimilar metals (or metal sites) that are electrically connected.
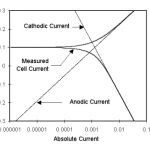
Figure 1-1. Corrosion Process Showing Anodic and Cathodic Current Components.
Figure 1-1 diagrams this process. The vertical axis is potential and the horizontal axis is the logarithm of absolute current. The theoretical current for the anodic and cathodic reactions are shown as straight lines. The curved line is the total current — the sum of the anodic and cathodic currents. This is the current that you measure when you sweep the potential of the metal with your potentiostat. The sharp point in the curve is actually the point where the current changes signs as the reaction changes from anodic to cathodic, or vice versa. The sharp point is due to the use of a logarithmic axis. The use of a log axis is necessary because of the wide range of current values that must be displayed during a corrosion experiment. Because of the phenomenon of passivity, it is not uncommon for the current to change by six orders of magnitude during a corrosion experiment.
The potential of the metal is the means by which the anodic and cathodic reactions are kept in balance. Refer to Figure 1-1. Notice that the current from each half reaction depends on the electrochemical potential of the metal. Suppose the anodic reaction releases too many electrons into the metal. Excess electrons shift the potential of the metal more negative, which slows the anodic reaction and speeds up the cathodic reaction. This counteracts the initial perturbation of the system.
The equilibrium potential assumed by the metal in the absence of electrical connections to the metal is called the Open Circuit Potential, Eoc. In most electrochemical corrosion experiments, the first step is the measurement of Eoc. The terms Eoc (Open Circuit Potential) and Ecorr (Corrosion Potential) are usually interchangeable, but Eoc is preferred.
It is very important that the Corrosion Scientist measures the Eoc and allows sufficient time for the Eoc to stabilize before beginning the electrochemical experiment. A stable Eoc is taken to indicate that the system being studied has reached “steady state”, i.e., the various corrosion reactions have assumed a constant rate. Some corrosion reactions reach steady state in a few minutes, while others may need several hours. Regardless of the time required, a computer-controlled system can monitor the Eoc and begin the experiment after it has stabilized.
The value of either the anodic or cathodic current at Eoc is called the Corrosion Current, Icorr. If we could measure Icorr, we could use it to calculate the corrosion rate of the metal. Unfortunately, Icorr cannot be measured directly. However, it can be estimated using electrochemical techniques. In any real system, Icorr and Corrosion Rate are a function of many system variables including type of metal, solution composition, temperature, solution movement, metal history, and many others.
The above description of the corrosion process does not say anything about the state of the metal surface. In practice, many metals form an oxide layer on their surface as they corrode. If the oxide layer inhibits further corrosion, the metal is said to passivate. In some cases, local areas of the passive film break down allowing significant metal corrosion to occur in a small area. This phenomena is called pitting corrosion or simply pitting.
Because corrosion occurs via electrochemical reactions, electrochemical techniques are ideal for the study of the corrosion processes. In electrochemical studies, a metal sample with a surface area of a few square centimeters is used to model the metal in a corroding system. The metal sample is immersed in a solution typical of the metal’s environment in the system being studied. Additional electrodes are immersed in the solution, and all the electrodes are connected to a device called a potentiostat. A potentiostat allows you to change the potential of the metal sample in a controlled manner and measure the current the flows as a function of potential.
Both controlled potential (potentiostatic) and controlled current (galvanostatic) polarization is useful. When the polarization is done potentiostatically, current is measured, and when it is done galvanostatically, potential is measured. This discussion will concentrate on controlled potential methods, which are much more common than galvanostatic methods. With the exception of Open Circuit Potential vs. Time, Electrochemical Noise, Galvanic Corrosion, and a few others, potentiostatic mode is used to perturb the equilibrium corrosion process. When the potential of a metal sample in solution is forced away from Eoc, it is referred to as polarizing the sample. The response (current) of the metal sample is measured as it is polarized. The response is used to develop a model of the sample’s corrosion behavior.
Suppose we use the potentiostat to force the potential to an anodic region (towards positive potentials from Eoc). In Figure 1-1, we are moving towards the top of the graph. This will increase the rate of the anodic reaction (corrosion) and decrease the rate of the cathodic reaction. Since the anodic and cathodic reactions are no longer balanced, a net current will flow from the electronic circuit into the metal sample. The sign of this current is positive by convention. For a discussion of electrochemical sign conventions, see the end of this document.
If we take the potential far enough from Eoc, the current from the cathodic reaction will be negligible, and the measured current will be a measure of the anodic reaction alone. In Figure 1-1, notice that the curves for the cell current and the anodic current lie on top of each other at very positive potentials. Conversely, at strongly negative potentials, cathodic current dominates the cell current.
In certain cases as we vary the potential, we will first passivate the metal, then cause pitting corrosion to occur. With the astute use of a potentiostat, an experiment in which the current is measured versus potential or time may allow us to determine Icorr at Ecorr, the tendency for passivation to occur, or the potential range over which pitting will occur.
Because of the range of corrosion phenomena that can be studied with electrochemistry, the ability to measure very low corrosion rates, and the speed with which these measurements can be conducted, an electrochemical corrosion measurement system has become a standard item in the modern corrosion laboratory.
The application note “Basics of Electrochemical Corrosion Measurements” is available at Gamry Instruments’ site in PDF format.